Radiation therapy of thoracic tumors, particularly lung carcinoma, is caught between the goals of maximizing tumor control probability and avoiding toxicities. Radiogenic pneumonitis is a relevant side effect. This occurs with a time latency of weeks to months after completion of treatment.
Radiation therapy of thoracic tumors, particularly lung carcinoma, is caught between the goals of maximizing tumor control probability and avoiding toxicities.
Radiogenic pneumonitis is a relevant side effect. This occurs with a temporal latency of weeks to months after completion of treatment, with the majority of cases appearing within the first 8 weeks post radiationem [1]. Clinical manifestations often include a symptom triad of exertional/dyspnea, nonproductive cough, and hypoxia, which contribute to the patient’s pronounced subjective sense of illness. Unlike bacterial or viral infections, febrile temperatures are uncommon; however, they may be secondary to superinfections. In the acute phase, CT morphology reveals a picture of interstitial edema. This acute inflammatory condition may subsequently heal either spontaneously or with drug intervention. However, in the long term, fibrosis of the affected lung tissue with consequent shrinkage is also possible. Depending on the extent of these in relation to the still functional lung, restrictive lung dysfunction may persist as irreversible residuals. Fortunately, fatal courses of the disease are rare and are reported in the literature to be less than 2% [2].
The underlying pathomechanism of radiopneumonitis is not yet fully deciphered in its complexity. The alveolar system is composed of type I and II pneumocytes. While type I pneumocytes, as differentiated cells, provide approximately 90% of the alveolar area, type II cells synthesize surfactant and serve as a repopulation pool for damaged type I cells [3]. Radiation-induced depletion of these cells results in loss of barrier function in the alveolus and reduction of microvascular integrity. These processes lead to edema formation and trigger, among other things, macrophage immigration and the release of proinflammatory cytokines [4]. Surviving pneumocytes can further differentiate into myofibroblasts and secrete transforming growth factor beta (TGF-beta), ultimately promoting the development of fibrosis.
Pneumonitis classification
The severity of pneumonitis is classified using the Common Terminology Criteria for Adverse Events (CTCAE), version 4.0: asymptomatic (grade 1); symptomatic, requiring medication, limiting activities of daily living (grade 2), severe symptoms, requiring oxygen, limiting activities of daily living (grade 3), or life-threatening respiratory dysfunction (grade 4). In clinical trials, the risk of grade 2 or 3 pneumonitis is most relevant.
The probability of occurrence of pneumonitis of any degree in primary definitive treatment of advanced lung cancer is reported to be 15% to 40%, depending on the literature source [1]. However, this therapy-related side effect also plays a role in the irradiation of other entities. A meta-analysis by Tonison et al. [5] identified, for example. 19 studies involving 874 patients who received radiotherapy for esophageal cancer. This showed a grade ≥2 pneumonitis rate of 6.6%. A retrospective analysis by Pinnix et al. [6] recorded pulmonary toxicity in 150 patients who underwent consolidative or salvage radiotherapy, including the mediastinum, for lymphoma. The stage-independent incidence of pneumonitis was 14%, with clinically relevant grades ≥2 occurring in 8% of patients. Also during adjuvant radiotherapy of breast carcinoma, relevant radiation exposure of the lung may occur, especially during elective irradiation of the mammaria interna lymph nodes parasternal. The EORTC 22922/10925 Trial [7] compared treatment outcomes of adjuvant radiation to the mammary gland or thoracic wall alone with additional treatment of the mammary internal and medial supraclavicular lymphatic drainage pathways. Analysis of toxicity showed an increased pneumonitis rate of 0.7% vs. 0.1% (p<0.0012) with extended irradiation. Although these values appear numerically low, it should be borne in mind that adjuvant radiotherapy for breast Ca is an absolute standard indication in many radiation oncology institutions. In Germany alone, 70,000 women are newly diagnosed with breast cancer each year, a large proportion of whom undergo irradiation as part of the therapy concept.
Dose-response relationships exist for both tumor control and radiogenic pneumonitis. Theoretically, especially large, locoregionally advanced bronchial carcinomas require a high radiation dose in the target volume, but its application is also associated with increased doses in healthy lung tissue and an increased risk of pneumonitis. Classically, radiation oncologists consult the data of the so-called QUANTEC (Quantitative Analysis of Normal Tissue Effects in the Clinic) review as a guideline value in the assessment of the safety of a radiation plan [8]. In this, the literature on therapy-induced radiation response on normal tissues was systematically reviewed. For the evaluation of the risk organ lung, it is recommended to limit the mean lung dose to 20 Gy in order to keep the rate of clinically manifest pneumonitis below 20%.
Risk with carboplatin/paclitaxel
However, in addition to such dosimetric guideline values, there are other patient- and therapy-related risk factors that are of relevance. In a comprehensive meta-analysis by Palma et al. [2], data from a total of 836 patients from 12 studies were included and analyzed for predictors of grade ≥2 penumonitis occurrence. All pa-tients received concurrent radiochemotherapy at diagnosis of non-small cell lung cancer (NSCLC) and the median applied dose was 60 Gy. The lung volume receiving ≥20 Gy (so-called V20) was identified as a relevant dosimetric parameter. This showed an OR of 1.03 per 1 percent increase in V20 (p=0.008). A significant increase in risk was demonstrated for combined simultaneous chemotherapy with carboplatin and paclitaxel (OR 3.33 vs. chemotherapy with cisplatin/etoposide, p<0.001). Regarding the age of the patients, there was only a trend for an increased risk (OR 1.24 per decade of life, p=0.09). However, multivariate analysis showed that the highest risk of developing pneumonitis (>50%) was in the combination of age >65 years and simultaneous carboplatin/paclitaxel administration.
In a modern randomized comparison of two dose levels for radiotherapy of non-small cell lung cancer (NSCLC), pneumonitis ≥ grade 3 occurred in 7% (therapy arm 60 Gy) and by 4% (therapy arm 74 Gy) [9]. The significantly worse survival in the high-dose arm is thus not explained by a documented higher rate of pneumonitis, but nevertheless suggests an influence of unrecorded treatment sequelae in combination with comorbidities.
Modern radiation techniques, especially intensity-modulated radiotherapy (IMRT) methods, allow a relevant reduction of the lung dose with a constant dose in the target volume compared to conventional 3D conformal planning. In a prospective phase 1 study by Boyle et al. [10] 24 patients were treated with definitive IMRT at diagnosis of advanced NSCLC and a corresponding radiation plan was calculated using 3D conformal technique for comparison. This showed a dosimetric advantage of IMRT both in terms of mean lung dose (11.9 vs. 14.9 Gy, p
Target volume planning based on 18F-fluorodeoxyglucose–(18F-FDG) PET/CT offers another option for dose reduction in healthy normal tissue. This procedure has become a standard for staging NSCLC and detects with high sensitivity the biological activity of a primarius, but also possible affected mediastinal lymph nodes. In conventional radiation planning, depending on the location of the tumor, the draining mediastinal lymph node stations are usually also electively irradiated, which sometimes results in large target volumes. Similarly, it is sometimes difficult to differentiate between primary tumor and consecutive atelectasis on conventional CT imaging (e.g., in airway obstruction), so that in cases of doubt, contouring is often somewhat more generous.
An Italian planning study [11] compared dose exposure in risk organs for 18 patients scheduled to receive primary radiotherapy for locally advanced NSCLC. Here, several individual treatment plans were created for each patient, including one version that included only the PET-positive structures and another version that included the elective lymph node levels. In 33% of patients, downstaging occurred based on PET imaging, particularly in that mediastinal Ln involvement could no longer be detected. By focusing the radiotherapy on the PET-positive foci, the median lung dose was reduced from a median of 20.5 to 15.5 Gy. The same applies to the V20, which fell from 34% to 26%.
In a prospective randomized trial, Nestle et al. [12], whether radiotherapy of PET-positive structures alone also leads to the same oncologic outcomes. A total of 205 patients with locally advanced NSCLC were randomized to either PET-based or conventional scheduling of primary radiochemotherapy. At a median follow-up of 29 months, no disadvantage of PET-based irradiation in terms of progression-free survival was demonstrated. The risk of local progression at one year was as low as 14% vs. 29% in the standard arm (HR 0.57, CI 0.3-1.06). It is thus safe to adapt the target volume for a patient individually on the basis of PET-CT and thus to reduce it significantly if necessary.
The ultimate question is whether the improvement in dosismetric parameters actually translates into a reduction in the clinical expression rate of pneumonitis. In the aforementioned dose comparison study by Bradley et al. [9], IMRT as well as the older 3D conformal technique was allowed in both arms. IMRT was used in patients with larger tumor target volumes (median 486 vs. 427 ml) but was associated with a lower rate of pneumonitis ≥ grade 3 (3.5% vs. 7.9%) and tended to have even better 2-year overall survival (53.2% vs. 49.4%) [13].
Reduction of safety margins thanks to gating
Another technical innovation in the field of radiotherapy is the implementation of gating procedures. By detecting and/or correcting the respiratory mobility of the tumor, these allow a reduction in the safety margins for the planning target volume (so-called PTV = Planning Target Volume), which takes into account daily positional inaccuracies in addition to the clinical extent of a primarius. Such an approach is used, for example, in This is made possible by performing the irradiation in deep inspiration, which is often referred to in the literature as DIBH (Deep Inspiration Breath Hold). To realize this, the patient’s breathing curve is digitally recorded via a spirometer or with surface scanners and made visible on a display. Patients are then prompted to take a deep breath via a visual or acoustic signal. If a previously defined threshold value is exceeded, the air is stopped in this position for about 15 seconds. Only during this time does the treatment device, the so-called linear accelerator, receive the go-ahead for irradiation, and patients can interrupt the therapy themselves at any time. After a short “breathing break”, the procedure continues until the end of the daily treatment session. After only a short familiarization phase, many patients are so familiar with the handling of the procedure that they breathe in independently at the right time above the threshold. So they learn, so to speak, to control the irradiation themselves with their own breath. A comparative study by Josipovic et al. [14] investigated the dosimetric differences between “free breathing” treatment and DIBH for 10 patients with advanced NSCLC and primary radiotherapy. Depending on the irradiation technique used, both the mean lung dose and the V20 could be significantly reduced by about 20% each by means of DIBH. In another analysis, the same research group addressed the question of whether patients with advanced NSCLC are suitable for treatment with respiratory arrest despite their underlying disease and possibly other pulmonary secondary diseases (e.g., COPD) [15]. In an interim analysis of a prospective study, you concluded that approximately 70% of the patients studied are able to perform a DIBH over 20 seconds and additionally benefit dosimetrically from this technique.
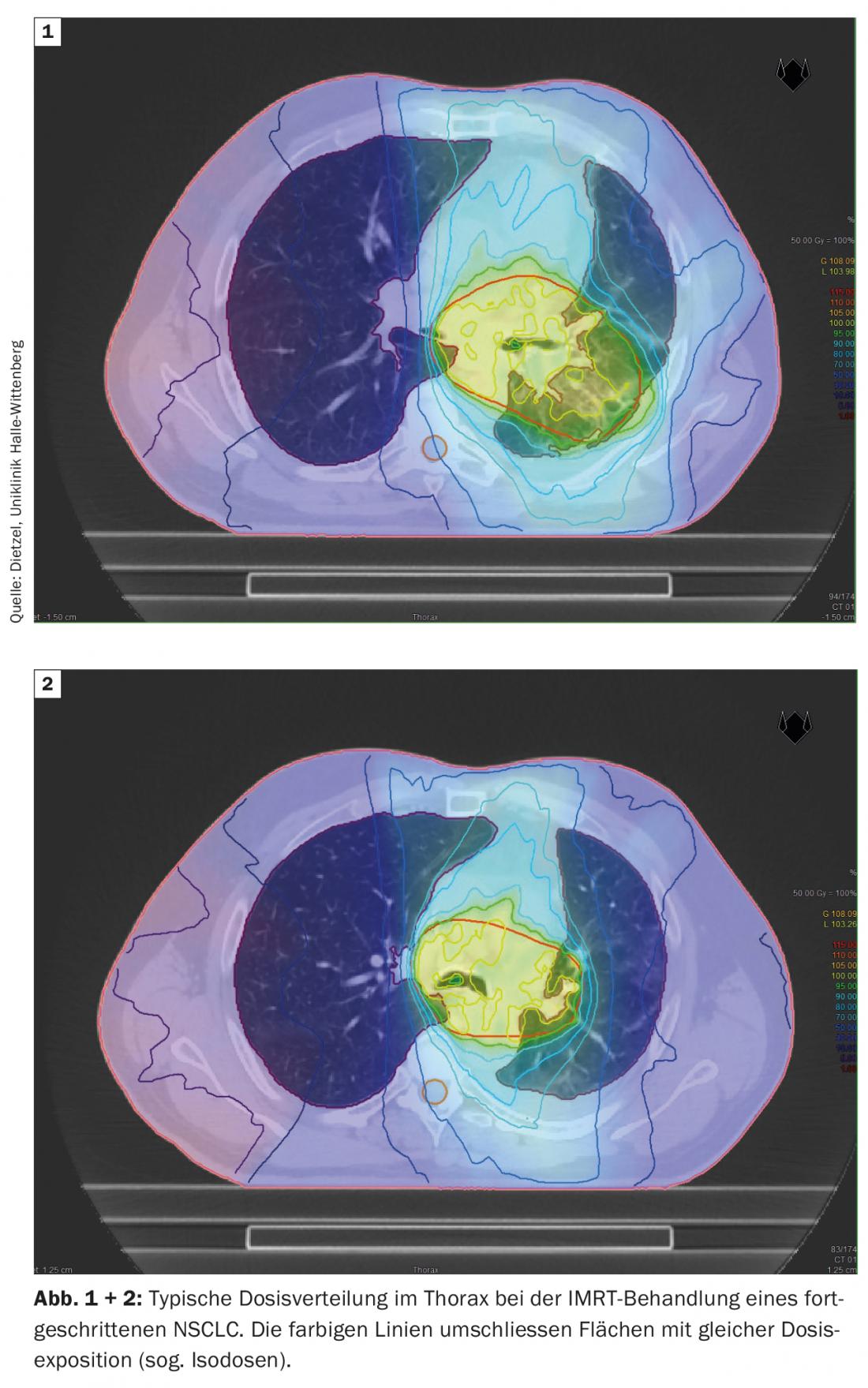
The DIBH technique also plays a decisive role in adjuvant radiotherapy of breast cancer, particularly in the case of left-sided tumor location, in order to avoid unnecessary radiation doses in the surrounding organs at risk. Here, the effect of deep insipration is mainly used to spare cardiac structures by lifting the thoracic wall away from the pericardium and lowering the heart caudally. However, as a positive side effect, the increase and shift in volume also protects the lungs. Based on a planning study at the Technical University of Munich considering 31 patients with mamma-Ca, it was shown that DIBH for the left-sided lung can reduce the mean dose by 19 ± 9% and the V20 by 24 ± 10% [16]. While large lung volumes are exposed to higher and intermediate doses in stage III NSCLC (Figs. 1 and 2), only small sections of the lung are exposed to relevant radiation doses during stereotactic radiotherapy of early-stage NSCLC (Fig. 3), which is why the method is used predominantly in internally inoperable patients – often with high-grade COPD and partial O2-obligation – is used. Even in this negatively selected group of patients, only rates of grade 2 and grade 3 pneumonitis of 7% and 2%, respectively, were observed with standard dose approaches [17].
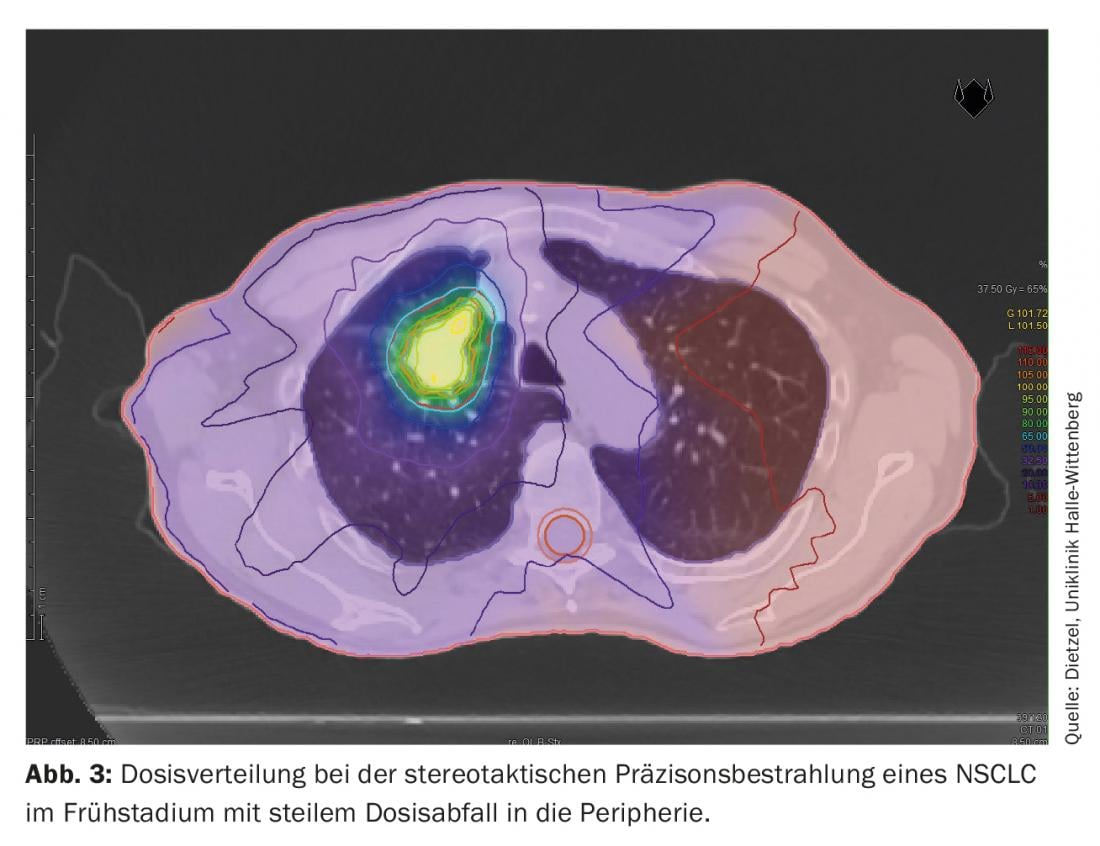
Immunotherapy opens up new perspectives
The introduction of immunotherapy in the treatment of NSCLC has opened new perspectives clinically in recent years, which have contributed to a significant improvement in prognosis. For example, for the sequential combination of radiochemotherapy followed by durvalumab as primary therapy, as studied in the PACIFIC trial. an improvement in 2-year survival from 55.6% to 66.3% (p=0.005) was demonstrated [18]. However, especially with regard to the additional toxicity of immunotherapy, well-founded long-term experience is currently only being gathered. In the evaluation of the PACIFIC trial, only a low rate of pneumonitis ≥ grade 3 of 3.4% was reported here, compared with 2.6% in the control arm (radiochemotherapy followed by placebo) [18]. In combination treatment of NSCLC from radiotherapy and immuno-oncology, pneumonitis should be considered as an overlapping toxicity. Initial data from a phase II study of simultaneous administration of nivolumab with radiochemotherapy showed a rate of grade 3 pneumonitis of 10.3%, considered acceptable by the authors but probably increased compared with radiochemotherapy alone [19].
Therapeutic options
According to the recommendations for the therapy of radiogenic pneumonitis of the current S3 guideline “Supportive Therapy in Oncological Patients” [20], treatment with steroids should be given. Typical dosing regimens include a daily prednisone dose of 60-100 mg/tgl. initial, which is slowly reduced over a period of 8-12 weeks [1]. This is a symptomatic therapy of the acute inflammatory phase of the disease, which presumably remains without influence on the later development of fibrosis [21]. Steroid administration may be supplemented or replaced by azathioprine or cyclosporine as decided on a case-by-case basis (e.g., if relief is insufficient). Prophylactic antibiotic administration is recommended only for patients with risk factors (e.g., immune suppression, stenosing processes, etc.). If fever is pronounced, calculated antibiotic therapy may be given. If this is ineffective, microbiological confirmation, e.g. via bronchial lavage, should be sought in order to initiate a resistogram-based therapy or to rule out fungal pneumonia. In drug prophylaxis, the administration of amifostine, which acts as a radical scavenger, is discussed. According to the guideline, the application can be performed as an off-label use in patients with planned radiochemotherapy. There is currently no effective treatment option for pre-existing fibrosis.
It remains to summarize that radiogenic pneumonitis is a relevant side effect of radiotherapy, which can lead to long-term patient distress via chronicity into pulmonary fibrosis. However, knowledge of relevant dose-response correlations and patient-related risk factors in combination with continuous technical innovation in the field of radiation oncology has led to the fact that one can speak of a calculable risk.
Take-Home Messages
- Radiogenic pneumonitis is a relevant side effect after irradiation of thoracic tumors and typically occurs after several weeks to months.
- In addition to an acute inflammatory phase, fibrosis with chronic concomitant symptoms may develop in the course of the disease.
- In addition to radiogenic lung exposure (mean lung dose and V20), patient-related factors (age, comorbidities) and the application of simultaneous systemic therapies also influence the probability of expression.
- Modern radiotherapy techniques such as intensity-modulated radiotherapy (IMRT), respiratory gating, and PET-based planning allow for a significant reduction in unwanted dose to the lung.
Literature:
- Jain V, et al: Radiation pneumonitis: old problem, new tricks. Cancers 2018; 10 (7).
- Palma DA, et al: Predicting radiation pneumonitis after chemoradiation therapy for lung cancer: an international individual patient data meta-analysis. Int J Radiat Oncol Biol Phys 2013; 85 (2): 444-450.
- Giuranno L, et al: Radiation-Induced Lung Injury (RILI). Front Oncol 2019; 9: 877.
- Citrin DE, et al: Role of type II pneumocyte senescence in radiation-induced lung fibrosis. J Natl Cancer Inst 2013; 105 (19): 1474-1484.
- Tonison JJ, et al: Radiation pneumonitis after intensity-modulated radiotherapy for esophageal cancer: institutional data and a systematic review. Sci Rep 2019; 9 (1): 2255.
- Pinnix CC, et al: Predictors of radiation pneumonitis in patients receiving intensity modulated radiation therapy for Hodgkin and non-Hodgkin lymphoma. Int J Radiat Oncol Biol Phys 2015; 92 (1): 175-182.
- Matzinger O et al: Toxicity at three years with and without irradiation of the internal mammary and medial supraclavicular lymph node chain in stage I to III breast cancer (EORTC trial 22922/10925). Acta Oncol 2010; 49 (1): 24-34.
- Bentzen, et al: Quantitative Analyses of Normal Tissue Effects in the Clinic (QUANTEC): an introduction to the scientific issues. Int J Radiat Oncol Biol Phys 2010; 76 (3 Suppl): 3-9.
- Bradley JD, et al: Standard-dose versus high-dose conformal radiotherapy with concurrent and consolidation carboplatin plus paclitaxel with or without cetuximab for patients with stage IIIA or IIIB non-small-cell lung cancer (RTOG 0617): a randomised, two-by-two factorial phase 3 study. Lancet Oncol 2015; 16 (2): 187-199.
- Boyle J, et al: Dosimetric advantages of intensity modulated radiation therapy in locally advanced lung cancer. Adv Radiat Oncol 2017; 2 (1): 6-11.
- Ceresoli GL, et al: Role of computed tomography and [18F] fluorodeoxyglucose positron emission tomography image fusion in conformal radiotherapy of non-small cell lung cancer: a comparison with standard techniques with and without elective nodal irradiation. Tumori 2007; 93 (1): 88-96.
- Nestle U, et al: Imaging-based target volume reduction in chemoradiotherapy for locally advanced non-small-cell lung cancer (PET-Plan): a multicentre, open-label, randomised, controlled trial. Lancet Oncol 2020; 21 (4): 581-592.
- Chun SG, et al: Impact of Intensity-Modulated Radiation Therapy Technique for Locally Advanced Non-Small-Cell Lung Cancer: A Secondary Analysis of the NRG Oncology RTOG 0617 Randomized Clinical Trial. J Clin Oncol 2017; 35 (1): 56-62.
- Josipovic M, et al: Deep inspiration breath hold radiotherapy for locally advanced lung cancer: comparison of different treatment techniques on target coverage, lung dose and treatment delivery time. Acta Oncol 2013; 52 (7): 1582-1586.
- Persson GF, et al: Deep Inspiration Breath Hold Compliance in Radiation Therapy for Locally Advanced Lung Cancer. Int J Radiat Oncol Biol Phys 2017; 99 (2): Supplement 2017: E491.
- Oechsner M, et al: Deep inspiration breath-hold for left-sided breast irradiation: analysis of dose-mass histograms and the impact of lung expansion. Radiat Oncol 2019; 14 (1): 109.
- Guckenberger M, et al: Is there a lower limit of pretreatment pulmonary function for safe and effective stereotactic body radiotherapy for early-stage non-small cell lung cancer? J Thorac Oncol 2012; 7 (3): 542-551.
- Antonia SJ, et al: Overall survival with durvalumab after chemoradiotherapy in stage III NSCLC. N Engl J Med 2018; 379 (24): 2342-2350.
- Peters S, et al: Safety evaluation of nivolumab added concurrently to radiotherapy in a standard first line chemo-radiotherapy regimen in stage III non-small cell lung cancer-The ETOP NICOLAS trial. Lung Cancer 2019; 133: 83-87.
- Oncology guideline program (German Cancer Society, German Cancer Aid, AWMF): Supportive therapy in oncology patients – long version 1.3, 2020, AWMF registry number: 032/054OL, www.leitlinienprogramm-onkologie.de/leitlinien/supportive-therapie; last accessed Aug. 24, 2020.
- Sekine I, et al: Retrospective analysis of steroid therapy for radiation-induced lung injury in lung cancer patients. Radiother Oncol 2006; 80 (1): 93-97.
InFo PNEUMOLOGY & ALLERGOLOGY 2020; 2(4): 6-10.