Wounds colonized with biofilms are one of the greatest challenges in chronic wound care. An estimated 2% of the population in Central Europe suffer from chronic wounds, with the risk increasing with age. Diagnosing and treating the underlying disease, usually peripheral arterial occlusive disease (PAVD), venous insufficiency (CVI), diabetes mellitus type I or II, or even an immunological disease, is always the first step to successful treatment. Recurrent (local) infections and persistent wound biofilm prolong the healing process.
Wounds colonized with biofilms are one of the greatest challenges in chronic wound care. An estimated 2% of the population in Central Europe suffer from chronic wounds, with the risk increasing with age. Diagnosing and treating the underlying disease, usually peripheral arterial occlusive disease (PAVD), venous insufficiency (CVI), diabetes mellitus type I or II, or even an immunological disease, is always the first step to successful treatment. Recurrent (local) infections and persistent wound biofilm prolong the healing process.
Wounds colonized with biofilms are one of the greatest challenges in chronic wound care [1,2]. An estimated 2% of the population in Central Europe suffer from chronic wounds, with the risk increasing with age [3]. Diagnosing and treating the underlying disease, usually peripheral arterial occlusive disease (PAVD), venous insufficiency (CVI), type I or II diabetes mellitus, or immunologic disease, is always the first step toward successful treatment [4]. Recurrent (local) infections and persistent wound biofilm prolong the healing process. This usually takes months and also requires multisectoral treatment (hospital, outpatient clinic, general practitioner or specialist, nursing) [5]. In addition, once wound closure is achieved, it does not guarantee that the wound will remain truly closed. Accordingly, the term “wound remission” is more appropriate than “wound healing” for patients with chronic wound healing disorders.
Wound biofilm – definition and pathogenesis
According to a meta-analysis, about 78% of all chronic wounds are colonized with pathogenic microorganisms in the form of biofilms [6]. These are, with the best possible therapy of the underlying disease, responsible for the chronicity of a wound [2]. Wound biofilm is defined as follows:
“Biofilm is a structured community of microbes with genetic diversity and variable gene expression (phenotype) that creates behaviors and defenses that result in the generation of unique (chronic) infections with significant tolerance to antibiotics and antimicrobials while being protected from host immunity.” [Consensus Delphi Process, IWII 05/2016].
Any wound, even an acute one, is colonized within a few hours by microorganisms of the skin microbiome, which may also contain pathogenic species. The wound bed is a good breeding ground for them. At this stage, one speaks of “wound contamination”. In the further course of time, the bacteria multiply in and on the wound; bacterial colonies form. External (antiseptic) wound cleansing can reduce bacterial colonization, and the immune system also works against the spread of bacteria in the wound. If these processes do not take place or if the local immune cells are not efficient enough, more and more bacteria colonize the wound. It is referred to as “colonized,” or “critically colonized” if the bacterial load increases with accompanying local reaction.
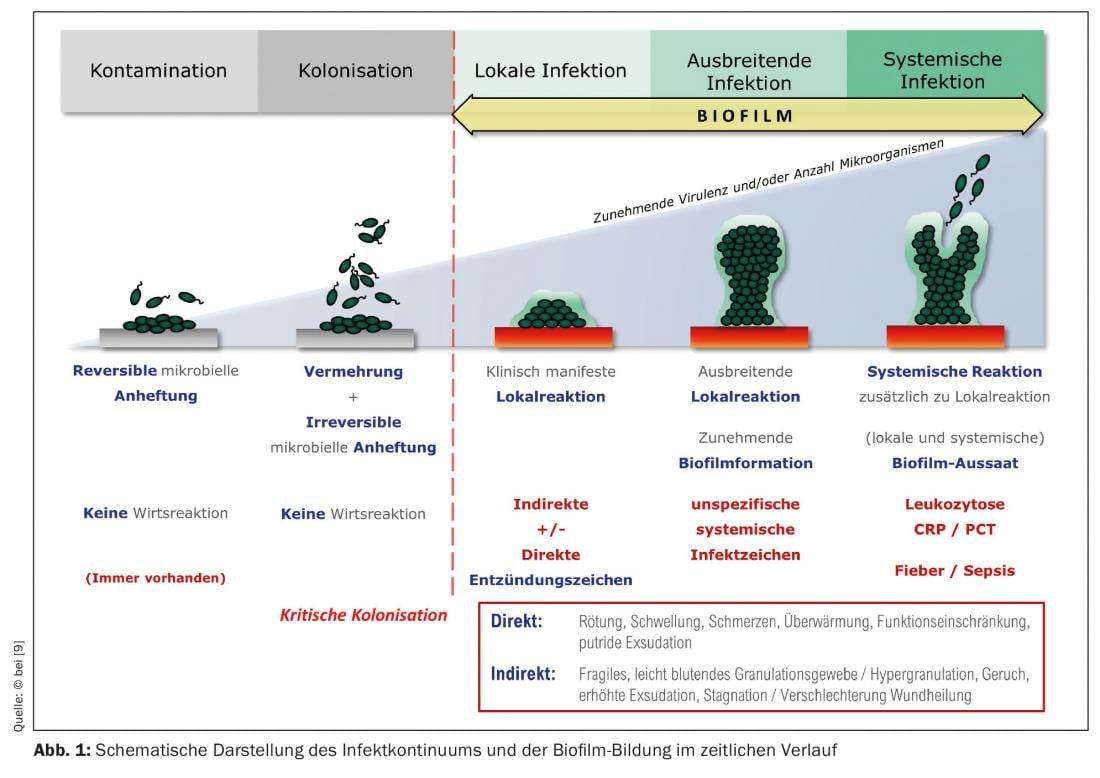
So-called immature biofilms can form from these bacterial colonies within only 24 hours (Fig. 1). In most cases, these biofilms do not consist of only one bacterial species (as is the case, for example, with implant biofilms in orthopedic surgery), but contain many different bacterial species, i.e. a multispecies biofilm. The leading bacterial species in wound biofilms, here exemplified by leg ulcers, are Staphylococcus aureus (47.6%), its methicillin-resistant variant (MRSA) (8.6%), Pseudomonas aeruginosa (31.1%), and Enterobacteriaceae (28.6%) [7]. These microorganisms – more rarely, fungi are also involved – begin to surround themselves with the so-called extrapolymeric substance (EPS), almost “walling themselves in” in it. EPS is mainly composed of polysaccharides (e.g., alginate, cellulose, dextran) and a variety of proteins, lipids, glycoproteins, glycolipids [8], i.e., sugars and proteins, which makes it adhere firmly to the wound bed. After 2-4 days, one speaks of “mature biofilm”. It can become up to 2 mm thick, thus plastic and visible (Fig. 2A/B). At this stage, the biofilm is able to release planktonic (suspended in fluid) bacteria with the wound exudate, which is produced abundantly due to inflammation (Fig. 1), which can colonize the wound environment or other wounds [9]. Here the cycle of biofilm formation begins again.
Detect wound biofilm
The first and most important step is the clinical diagnosis, i.e. to even consider the possibility of biofilm colonization of the (chronic) wound as an interfering factor in healing. The mature biofilm can be easily identified and verified clinically by wound (edge) exploration using forceps or curettes (Fig. 2A/B) . The wound biofilm itself provides locally for a permanent, more or less pronounced immune response or inflammation. It can be the basis of a wound infection, but can also persist for weeks and months without inducing it.
In the case of wounds with bare biofilm persistence, there is usually no pronounced (tissue) damage, apart from the lack of healing, since the various microbial species exist symbiotically in the biofilm with reduced metabolism and reduced proliferation and feed on (human) wound exudate. On the other hand, there are also highly aggressive wound biofilms, e.g. those dominated by P. aeruginosa , which lead to severe exudation and environmental dermatitis, consequently causing wound enlargement. An accompanying strong wound odor is common.
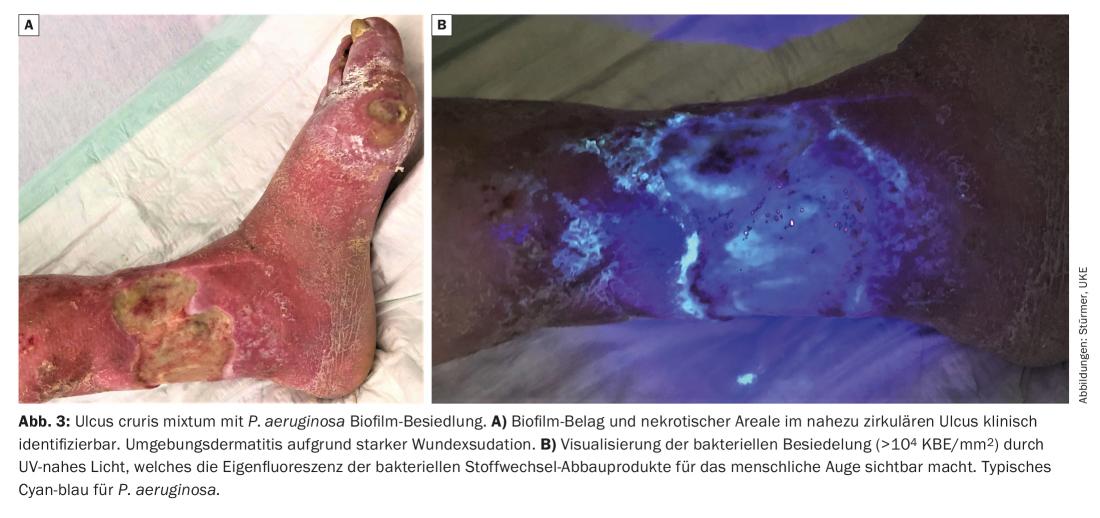
A wound biofilm or a strong bacterial colonization of the wound (>104 CFU/mm2) can be made visible to the human eye using UV-near light (e.g. MolecuLight®, MolecuLight Corp., Toronto, Canada) (Fig. 3) : Using light of wavelength 450 nm, areas of high bacterial metabolic activity can be visualized by the red fluorescence of deposited bacterial metabolites, such as porphyrins (from e.g. Staphylococcus Spp. and Enterobacteriaceae) or by the cyan-blue fluorescence of pyoverdine secreted by Pseudomonas Spp. [10]. Thus, it could also be detected on the basis of many wounds that P. aeruginosa predominantly colonizes and infiltrates the wound margin, whereas S. aureus is more likely to be found at the wound base.
The therapy of the wound biofilm
The symbiotic, interspecies community of microorganisms from which biofilms are formed produces a protective, so-called extrapolymeric substance (EPS) that functions as a biochemical barrier against the host immune system and, in particular, against antimicrobial agents [11,12]. Therefore, the latter often fail in biofilm eradication [13–15] (Fig. 4) . In addition, signaling molecules allow microorganisms to communicate with each other, e.g. to change their metabolic activity [13,15]. Lower metabolic activity of specific bacteria, the so-called persister cells in the depth of the biofilm, as well as the interaction of different microorganisms, e.g. by lateral resistance gene transfer, are further aspects contributing to the high resilience of biofilms [17]. Its “tolerance” to antimicrobial substances, solutions and dressings is high, as these lead to the induction of cell death of bacteria and fungi, but not to the destruction of EPS. Thus, the antimicrobial agents cannot develop their efficiency against microorganisms, since – due to the “protective shield” of the EPS – they do not even penetrate to them [15]. The same applies to systemically applied antibiotics, which, due to EPS, cannot penetrate deeply enough into the wound biofilm from the inside, i.e. from the wound bed, to completely eliminate the pathogenic germs. Not only for this reason, but in general, the prescription of antibiotics in the therapy of chronic wounds should be critically considered in order to avoid the development of resistance in the long healing process of chronic wounds.
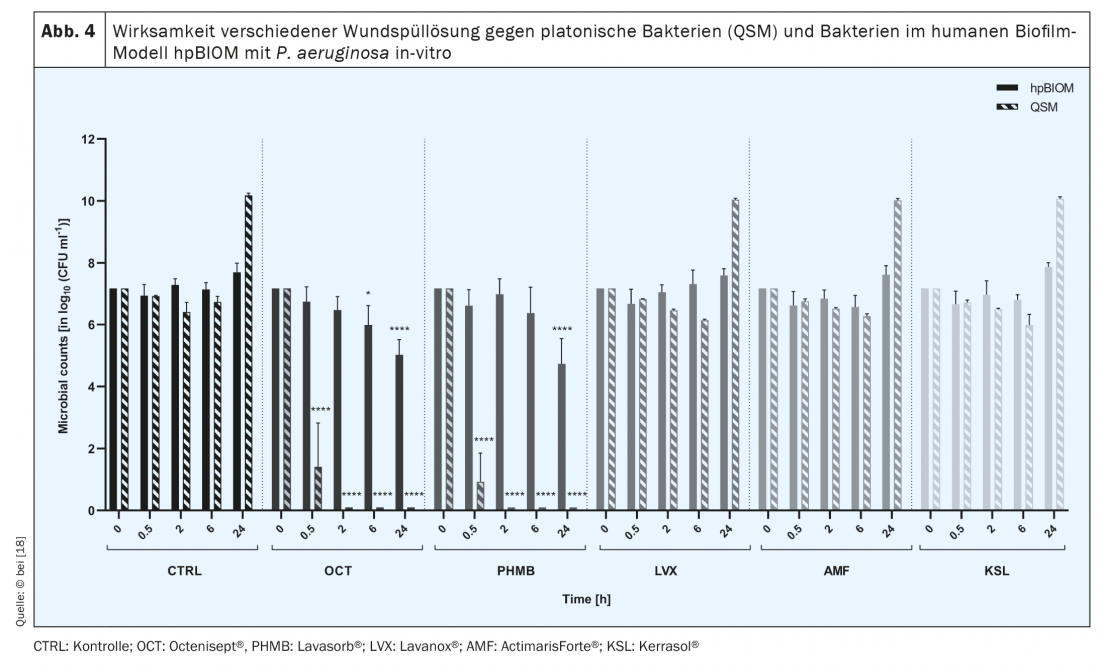
Wound biofilm and (antimicrobial) wound irrigation solutions
There are several approaches to eliminate biofilm colonization of wounds [17]. For example, commercially available antimicrobial solutions that have been shown to be effective in in vitro analyses are recommended. However, clinical observation sometimes contradicts the reported antimicrobial performance [18] (Fig. 4).
There are different types of wound irrigation solution: in the case of some, such as physiological saline solution or NaCl, the focus is on the cleansing, diluting effect; in the case of other groups, on the more or less pronounced antimicrobial efficacy. It is known that some wound irrigation solutions lose their antimicrobial potential when they come into contact with protein-rich environments, such as those of wound exudate [19]. Accordingly, the effectiveness of different wound irrigation solutions against bacterial biofilms also varies, with the EPS also acting as a “protective shield”. Antimicrobial wound irrigation solutions address and destroy bacterial walls, membranes and bacterial transport proteins or inhibit their function. If they do not succeed in breaking through the EPS, which consists mainly of polysaccharides and proteins, they cannot act effectively.
Comparative clinical studies with (antimicrobial) wound irrigation solutions, which could be the basis for differentiated therapy recommendations, do not exist. However, systematic translational testing using the human biofilm model hpBIOM shows that Octenisept® is able to cleave biofilms and kill the bacteria within them within 72 h (!). [15,18] (Fig. 5). PHMB succeeds to a limited extent in the same period, but complete eradication is not achieved even after 72h. The hypochlorous wound irrigation solutions and chlorhexidine fail to effectively eliminate bacteria in biofilm [18].
Wound biofilm and antimicrobial dressings
Wounds colonized by biofilm can basically be divided into two types: Wounds with resident, less aggressive biofilm. These are characterized by a quiet wound environment with light to moderate exudation (Fig. 2) . The other wound biofilm type shows heavy exudation, which requires daily dressing changes. Accompanying swelling, redness, a high sensitivity of the entire wound region and often even an environmental dermatitis are obligatory (Fig. 3). Thus, in these patients, special attention should also be paid to the surrounding skin of the wound and it should also be treated (e.g., urea, zinc or low-dose cortisone-containing ointments).
Comparative clinical studies with (antimicrobial) wound dressings are scarce [20]. It is difficult to derive differentiated therapy recommendations from this, since not only the active substances (silver ions, nanocrystalline silver, PHMB, iodine derivatives, etc.) and their concentrations vary, but also the respective basis of the wound dressings (PU foam, alginates, fibers, etc.). In multiple, own in vitro tests using a human biofilm model, a combination product of iodine and starch (Cardexomer-Iodine) was able to completely eradicate the bacteria in the biofilm within 6 (!) days (Fig. 5). Other wound dressings containing active ingredients with PHMB or silver (MP class 2B) reduced the bacterial load only [21]. However, the antimicrobial dressings were analyzed over 6 days without change; higher effectiveness should be achieved by all dressings tested with daily or 2-day dressing changes.
Wound biofilm and (sharp) debridement
Consistent sharp or surgical debridement is currently the only effective and sustainable therapy for wound biofilm recommended by expert consensus [6]; however, it is not always a viable option in the home-care setting (e.g., limited hygiene, drug-treated-anticoagulated patients). Applying this comparatively aggressive local therapy even to “calm” wounds with biofilm colonization (Fig. 2) sometimes requires some effort on the part of the practitioner: After dressing removal, the wound should be inspected for potential biofilm colonization visually or with the aid of the above-mentioned UV light, in order to remove it using a curette and/or scalpel if necessary. Only after its removal can antimicrobial substances in solutions and wound dressings act effectively against the remaining pathogenic microorganisms.
Surgical debridement is often indicated when there is a need to remove necrotic tissue in addition to the wound biofilm [22]. It is performed under conduction or intubation anesthesia. Compared to sharp debridement, it is more invasive and also removes parts of the wound margin and wound bed using a scalpel and shaver. The size of the wound increases accordingly at first. Another option for removing wound biofilm is chemical debridement (e.g., Debrichem®), which effectively eliminates bacteria but also infiltrates the wound bed and wound margin. Here, deep-seated bacteria are killed, but human cells are also involved, so that here, too, the primary effect is wound enlargement [23,24].
Conclusion
The (bacterial) wound biofilm requires complex therapy locally because it consists of several “components”. It is important to realize that it is found on more than 75% of all chronic wounds and thus can be removed. Wound cleansing by means of irrigation solution and compress alone is not sufficient. The persistence of biofilm colonization causes wound healing to stagnate. Regardless of the local therapy, however, the underlying disease that led to the chronification of the wound must always be treated causally. Accordingly, a biofilm-populated, heavily exuding wound is also not a contraindication for medically indicated compression therapy (e.g. in CVI, lymphedema or lipedema).
Take-Home Messages
- Diagnosing and treating the underlying condition is always essential in patients with chronic wounds.
- Around 78% of all chronic wounds are colonized with pathogenic microorganisms in the form of biofilms.
- The leading bacterial species in wound biofilms are S. aureus and P. aeruginosa.
- Antimicrobial wound irrigation solutions and dressings address and kill bacteria. However, they often do not penetrate the EPS of the biofilm, which consists mainly of polysaccharides and proteins, and thus cannot act effectively.
- Consistent sharp or surgical debridement is currently the only effective and sustainable therapy for wound biofilm recommended by expert consensus.
Literature:
- Noskin GA, Rubin RJ, Schentag JJ, et al: National trends in Staphylococcus aureus infection rates: impact on economic burden and mortality over a 6-year period (1998-2003). Clin Infect Dis 2007; 45(9): 1132-1140.
- James GA, Swogger E, Wolcott R, et al: Biofilms in chronic wounds. Wound Repair Regen 2008; 16(1): 3-44.
- Heyer K, Herberger K, Protz K, et al: Epidemiology of chronic wounds in Germany: analysis of statutory health insurance data. Wound Repair Regen 2016; 24(2): 434-442.
- Hachenberg T, Senturk M, Jannasch O, Lippert H: [Postoperative wound infections. Pathophysiology, risk factors and preventive concepts]. Anaesthesist 2010; 59(9): 851-866; quiz 867-858.
- Augustin M SrE, Dissemond J, Gerber V, et al: Recommendations to improve the structure of care for people with chronic wounds in Germany. Wound Management 2020; 14: 357-365.
- Malone M, Bjarnsholt T, McBain AJ, et al: The prevalence of biofilms in chronic wounds: a systematic review and meta-analysis of published data. J Wound Care 2017; 26(1): 20-25.
- Jockenhofer F, Gollnick H, Herberger K, et al: Bacteriological pathogen spectrum of chronic leg ulcers: results of a multicenter trial in dermatologic wound care centers differentiated by regions. J Dtsch Dermatol Ges 2013; 11(11): 1057-1063.
- Flemming HC, Wingender J.: The biofilm matrix. Nat Rev Microbiol 2010; 8(9): 623-633.
- Rembe JD, Stuermer EK: Modern wound antisepsis – indications and limitations, between knowledge, desire and uncertainty. Vascular Surgery 2020; 25: 272-276.
- Stuermer EK, Besser M, Debus ES, et al: Bacterial infiltration in biofilm-colonized wounds: Analyses in the hpBIOM ex vivo wound model and possible impact on swabbing and debridement. Int Wound J 2022 (under revision).
- Thurlow LR, Hanke ML, Fritz T, et al: Staphylococcus aureus biofilms prevent macrophage phagocytosis and attenuate inflammation in vivo. J Immunol 2011; 186(11): 6585-6596.
- Cowan T: Biofilms and their management: from concept to clinical reality. J Wound Care 2011; 20(5): 220, 222-226.
- Larsen T, Fiehn NE.: Resistance of Streptococcus sanguis biofilms to antimicrobial agents. APMIS 1996; 104(4): 280-284.
- Percival SL, Salisbury AM, Chen R: Silver, biofilms and wounds: resistance revisited. Crit Rev Microbiol 2019; 45(2): 223-237.
- Besser M, Dietrich M, Weber L, et al: Efficacy of antiseptics in a novel 3-dimensional human plasma biofilm model (hpBIOM). Sci Rep 2020; 10(1): 4792.
- Williams P, Winzer K, Chan WC, Camara M: Look who’s talking: communication and quorum sensing in the bacterial world. Philos Trans R Soc Lond B Biol Sci 2007; 362(1483): 1119-1134.
- Johani K, Malone M, Jensen SO, et al: Evaluation of short exposure times of antimicrobial wound solutions against microbial biofilms: from in vitro to in vivo. J Antimicrob Chemother 2018; 73(2): 494-502.
- Rembe JD, Huelsboemer L, Plattfaut I, et al: Antimicrobial Hypochlorous Wound Irrigation Solutions Demonstrate Lower Anti-biofilm Efficacy Against Bacterial Biofilm in a Complex in-vitro Human Plasma Biofilm Model (hpBIOM) Than Common Wound Antimicrobials. Front Microbiol 2020; 11: 564513.
- Rembe JD, Fromm-Dornieden C, Stuermer EK: The influence of human acute wound fluid (AWF) on the antibacterial efficacy of different antiseptic polyurethane foam dressings: an in vitro analysis. Wound Repair Regen 2018; 26: 27-35.
- Schwarzer S, James GA, Goeres D, et al: The efficacy of topical agents used in wounds for managing chronic biofilm infections: A systematic review. J Infect 2020; 80(3): 261-270.
- Stuermer EK, Plattfaut I, Dietrich M, et al: In vitro activity of antimicrobial wound dressings on P. aeruginosa wound biofilm. Front Microbiol 2021; 12: 664030.
- Dissemond J, Bültemann A, Gerber V, et al: Position paper of the Initiative Chronische Wunde (ICW) e.V. on the nomenclature of debridement of chronic wounds. Dermatologist 2022; 24 [Online ahead of print].
- Schwarzer S, Radzieta M, Jensen SO, Malone M: Efficacy of a topical wound agent methane-sulfonic acid and dimethylsulfoxide on In Vitro Biofilms. Int J Mol Sci 2021; 22: 9471.
- Cogo A, Quint BJ, Bignozzi CA: Restarting the Healing Process of Chronic Wounds Using a Novel Desiccant: A Prospective Case Series. Wounds 2021; 33: 1-8.
HAUSARZT PRAXIS 2022, 17(7): 12-17